How many times have you been caught with low battery power on your mobile and no charger? ‘Zero-power communications’ means devices can be battery-less and cordless because they are powered, quite literally, ‘out of thin air’. While some time off (if ever) for complex devices like mobiles, zero-power communications is on the verge of revolutionising the Internet of Things (IoT), according to a recent while paper from a leading Chinese equipment vendor, Oppo.
When ‘batteries included’ is a problem for IoT
The Oppo paper notes that while advances in IoT devices have driven towards lower power consumption and lower costs, the need for an inbuilt power source (i.e. a battery) can be a constraint on the ubiquity of IoT, for the following reasons.
IoT devices are often intended to be left in place for a long time, such as remote climate or soil monitoring devices ‘seeded’ across harsh external environments or sensors ‘buried’ in the fabric of buildings to support preventative maintenance. But ‘set and forget’ is only as good as the battery life, and repairing or replacing batteries (or seeding out replacement devices) across a dispersed, difficult access fleet of IoT devices can be expensive.
Other potential uses of IoT require devices of an ultra-small scale, which is hard to achieve with a battery onboard. For example, an IOT terminal used for food chain management in the form of electronic label on packaging.
Wearable devices using battery powered IoT has drawbacks: the batteries can be bulky on or in the garment, batteries don’t take kindly to being washed or dry cleaned, and periodically needing to plug in your cardigan or scarfs is inconvenient.
There are some environments in which battery-powered devices are potentially impractical. Many industrial sensor nodes may need to operate in environments where conventional batteries are not suitable because of the requirements of the physical and chemical characteristics of the battery: e.g. in high/low temperatures, or attached or adjacent to high-speed moving or rotating parts, such as aircraft engines.
A rapidly expanding use of IoT is for in-body monitoring and scanning. Injecting or ingesting battery-powered devices into the human body can be potentially dangerous.
Lastly, batteries (and the transmitters they power) add to the cost of IoT devices. Lowering IoT device costs will enhance competitiveness with alternative technologies, such as bar codes.
So, how can IoT do without batteries?
Zero-power communication technology utilizes two key radio techniques:
- RF energy harvesting: transmitters, such as mobile base stations, use radiated power in propagating electromagnetic waves. RF energy harvesting converts radio frequency energy into direct current (RF-DC). The energy can be stored in a unit such as a capacitor or it can be directly used to drive sensors, logic circuits and digital chips.
- Backscattering communication: this allows an IoT device to transmit data by reflecting and modulating an incident RF wave (i.e. transmitted by another source which the IoT device picks up). In a way, backscattering communication is similar to radar technology, a part of electromagnetic waves will be reflected when they reach the surface of an object, but the difference being that the IoT device will reshape or ‘modulate’ the reflected transmission to carry the data the IoT device has collected (essentially, the outbound transmission ‘piggy backs’ on the reflection of the inbound transmission). This means that the IoT device does not need active RF components and as a result can be made to have miniature hardware with extremely low power consumption.
The most obvious source of ‘incident’ RF waves for RF energy harvesting and backscattering is the transmitter in the network to which the IoT device is connected, such as a mobile tower. However, backscatter devices can be ‘omnivorous’: they can communicate with each other by using harvested power and signals transmitted from any ambient RF sources such as TV towers, FM towers, cellular base stations, and Wi-Fi access points.
The Oppo report describes the cumulative impact of these techniques (plus low power computing) in the following terms:
“..in terms of power consumption, the terminal power consumption is expected to be reduced from tens of milliwatts of NB-IoT terminals to dozens of microwatts or even several microwatts. In terms of cost, the terminal cost is expected to be reduced from more than ten RMB yuan [A$2.00] of the cheapest NB-IoT terminal in the above technologies to 1 RMB yuan [$A0.21] or even lower.”
Folding Zero-powered IoT into existing radiocommunications networks
While zero-power communications devices can use unlicensed or class licensed spectrum, the Oppo paper identifies distinct advantages in using them within conventional mobile networks:
- “the coverage of the zero-power communication network is primarily limited by the coverage of the wireless power in the forward link, that is, the forward link is a coverage bottleneck”: in other words, because the inbound transmission is the sole source of power for the IoT device, the better the inbound transmission, the better the IoT device’s performance can be. Mobile networks tend to produce more powerful, reliable RF signals for IoT devices to harvest power and backscatter signals because the networks utilise powerful transmitters to ‘pump out’ RF signals over wide areas, with capacity to support many users and the ability to manage interference between the hosted devices.
- mobile networks also tend to utilise a range of spectrum, including low band spectrum (e.g. 700MHz) which improves the backscattering maximum transmission distance: in the frequency band of 2.4GHz, the maximum backward link distance is 176m but when 700MHz frequency band is used, the maximum backward link communication distance increases to 606 m;
- a mobile based zero-power communication system can support the large-scale deployment and centralised control of zero-power terminals, which can greatly improve the system efficiency and save the deployment cost compared with the existing methods based on QR code or RFID.
The following diagram from the Oppo report illustrates how zero-powered IoT could be folded into a mobile network:
Case 1: the simplest zero-power communication, in which the mobile base station and the zero-power IoT device deal directly with each other. The base station provides wireless power sourcing signal and trigger signal to the zero-power terminal. The wireless power sourcing signal is used to provide energy to the zero-power terminal. The trigger signal carries the control information. In response to the control message, the zero-power terminal uses backscattering of the inbound signals to transmit the information to the base station.
Case 2: a zero-power terminal is combined with traditional device to achieve energy saving in the traditional device. When the base station needs to communicate with the traditional device, it first sends a wake-up signal, and the zero-power terminal wakes up the traditional device when detecting the wake-up signal. The traditional device then transmits the data it has collected to the base station. This achieves the ‘best of both worlds’: use of the zero-power IoT as the wake-up can save the power consumption consumed for traditional paging monitoring, and once woken up, the traditional device is able to utilise its much superior transmitting and other RF functions compared to what is achievable with the zero-powered device.
Case 3: while mobile networks may ‘pump out’ more RF energy, the energy which can be harvested ‘drops off’ the further and more scattered the zero-powered devices are throughout the cell area. A zero-power terminal can obtain wireless power supply not only from the base station communicating with it, but also from other network equipment or the third-party equipment. Wireless power supply from more nodes can significantly improve the coverage. Potential power supply nodes include smart phones, relay nodes, CPE, etc. If necessary, dedicated power supply nodes can also be deployed. The traditional wireless communication signals (such as synchronization signal, broadcast signal, data channel, etc.) sent by these nodes can be used to provide wireless power for zero-power terminals, or these power supply nodes can also send specific wireless power supply signals. In effect, the zero-powered IoT devices can be used for the ‘last mile’.
But there are limits to pulling energy from ‘thin air’
The first limitation is transmission distance. The standard coverage is around 100ms, which works well in a factory setting but not for IoT ‘seeded’ over much wider areas, such as in a rural setting. The distance limitation is not so much the backscattering distance (i.e. the distance over which the IoT can reflect signal) but the forward distance (i.e. the maximum distance over which the IoT device can harvest energy). While the maximum backscattering distance when using 700MHz is over 600 ms, the maximum forward signal distance is only 68ms. The forward distance can be increased by using high-gain antennas at the transmitting site (e.g. the base station), but paradoxically, that substantially reduces the backscattering distance. Hence, in many settings, case 3 above where the zero-powered IoT devices ‘hang off’ powering nodes scattered through the network.
Second, while making the zero-powered devices much less sophisticated RF devices reduces the power requirements, it creates its own complications. In traditional mobile systems, flexible resource allocation between the end user device and the base station (e.g. using multiple spectrum bands) can meet the requirements of different service type with diverse peak rate, latency and so on. But for zero-power terminals with extremely low cost and complexity, the flexibility of resource allocation that they can support will be greatly reduced because they are so simplified RF devices.
The Oppo paper gives the example of synchronisation. In a traditional mobile communication system, in order to reduce the interference between multiple users and the interference between uplink and downlink in TDD system, there needs to be tight, very exact requirement of synchronization between the network and the end user device. Traditional devices, such as smartphones, Machine Type Communication devices and other IoT devices, can meet the synchronization requirements of mobile systems. If a zero-power terminal is to be ultra-low cost and complexity, it can only be equipped with a simple oscillator of small size and low power consumption, such as RC oscillators, to provide the clock. However, the accuracy of RC oscillators is poor, with errors up to 1% or even higher.
This is not Sci-fi
Many modern implantable medical devices (IMDs), such as cardiac pacemakers, have wireless communication capability which allows medical professionals (or patients) to externally monitor the device’s performance.
There is an increasing concern that IMDs are vulnerable to attack, including a ‘battery depletion attack’ or battery-DoS. As the radio transmitter has to draw on the same battery power as the pacemaker function, the transmitter typically goes into ‘sleep mode’ until ‘woken up’ by an external signal, such as from the treating doctor’s tablet. A battery-DoS involves interfering with the ‘wake-up/sleep modes’, such as by speeding them up into a continuous cycle, to deplete the battery. The cardiac pacemaker can be fairly readily ‘hacked’ by anyone standing nearby the patient.
There are proposals to use zero-powered device incorporated into the pacemaker to be the ‘gatekeeper’. The device would use the inbound signal (from a legitimate or illegitimate source) to power security protocols and checking. Only once it was satisfied that the signal was from a legitimate source would it ‘wake up’ the transmitter. This would avoid the nefarious signals running down the battery power.
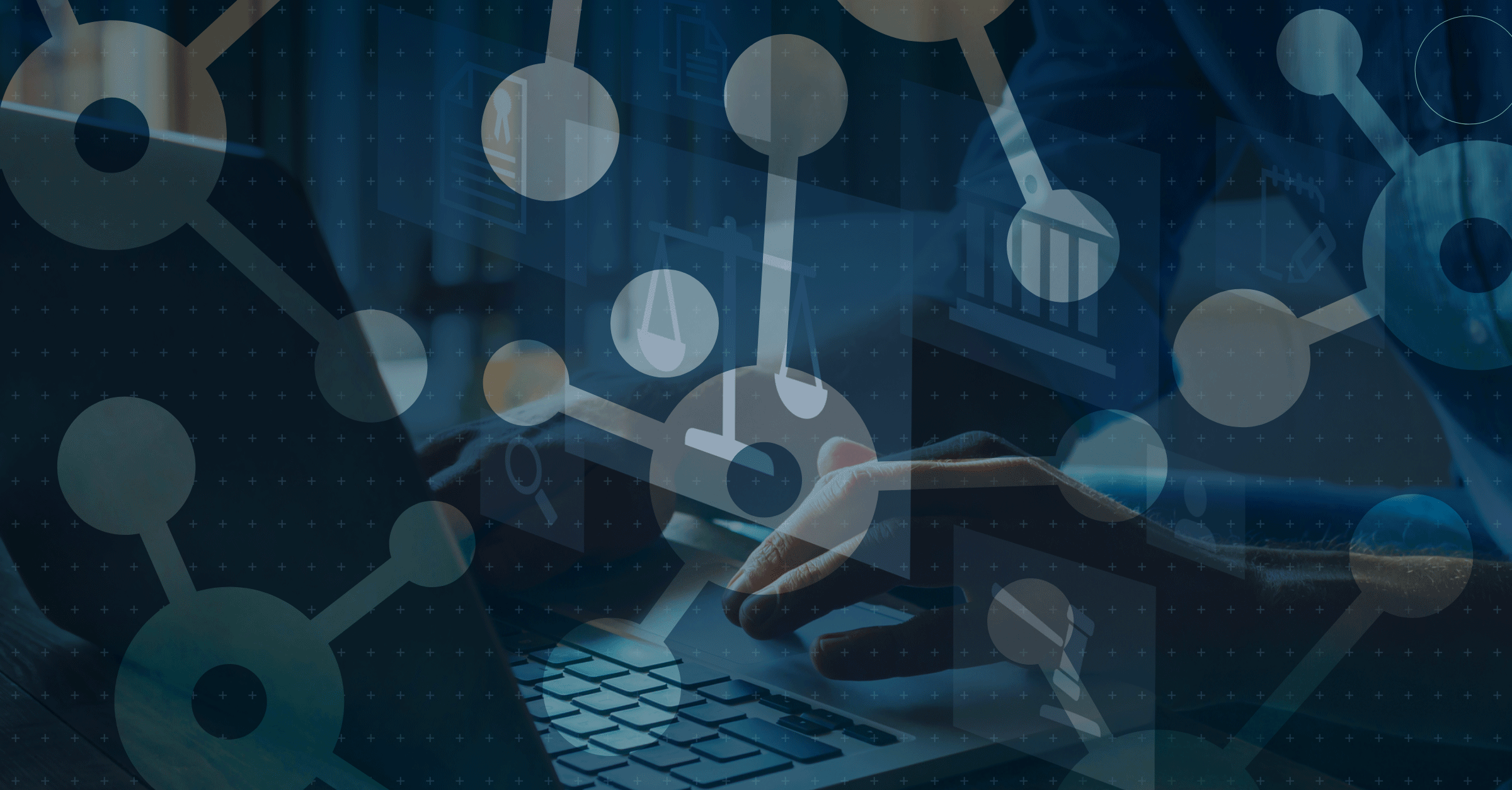
Visit Smart Counsel