We are truly experiencing a quantum shift in the way we get around.
The role of the internal combustion engine (ICE) has served us for over a century, as we have both worked and played. Fuel for the ICE has contributed to huge exploration and production efforts, both on land and at sea, as we searched for and produced from, new reserves. It wasn’t that long ago that many experts were predicting the world would reach “peak-oil” after which, the doomsayers were describing a fall in the world’s economic activity with the consequential lowering of our core standard of living. More recently, these concerns were overcome by us demonstrating our ability to extract hydrocarbons from the tight formations, using both horizontal drilling techniques and fracking technology, both of which are expected to result in the United States being a net energy exporter by 2022.
Against this background of the Western world enjoying a new energy security, we are witnessing the rise of the electric vehicle (EV). Not only is this revolutionising the transport industry, the battery technology which is being developed to support the EV is awakening the opportunity for battery technology to be used more broadly for energy storage. This will see both homes and industry sourcing their power needs off the grid.
When buying an EV, consumers need to be conscious of the impact charging the battery will have on the electricity demand in their household where charging an EV may double electricity demand. It is because of this that in parallel with the growing demand for EV’s, society will need to provide charging stations or individual households will need to take an integrated approach to their energy needs by installing solar or unit generating capacity and then storing the generated power in a domestic battery system. The simple fact is the existing distribution grid may not be able to service the extra load required to charge the expected EV take-up.
Although beyond the scope of this article, the emergence of the EV is likely to see grid operators having to become more active with their data management, as they look to see the grid utilised more during the off-peak periods. Changing infrastructure will therefore be a key factor in the adoption of EV’s, along with the reduction of the cost of an EV and an increase in the EV driving range.
UBS has undertaken some very informative analysis on EV’s. In May 2017, UBS released a paper which reported on the tear down of what was the world’s first mass-market EV, the Chevy Bolt. This vehicle was compared with what UBS considered was the equivalent ICE car, the VW Golf. The findings of this tear down make for some very interesting reading, namely:
- currently, the battery pack in the Bolt is the largest cost item in that car;
- the cost of the battery pack is expected to reduce by 36% by 2025E;
- total ownership costs between EV’s and ICE cars should reach parity in 2023E in Europe and 2025E in China; and
- forecast EV sales are expected to be 3.1 million in 2021E and 14.2 million in 2025E.
UBS produced in its analysis an estimated cost breakdown between the Bolt and Golf which is reproduced below:
To appreciate the cost differences between the two vehicles, UBS prepared a comparison between the number of moving parts in the engine of each vehicle.
Due to the role of the battery in an EV, the Bolt is heavier than the Golf. Again, the UBS analysis provided a very useful comparison.
It is the role of the battery in an EV which is what has set the resources sector alight. UBS compared the weight of the resources which are used in the Bolt when compared to those in the Golf and found the Bolt contained:
- “70% more aluminium;
- 80% more copper;
- 7% less steel;
- 60% less iron;
- 100% less precious metals;
- 140kg of “active” materials in the battery cells (Nickel, Cobalt, Lithium, Manganese, Graphite);
- 1kg of rare earths in the e-motor, in particular neodymium and dysprosium; and
- the same amount of rubber”.
The battery used in the Bolt is produced by LG Chem of Korea and percentage weight of the minerals in the battery is demonstrated in the following diagram:
What is obvious though is that as time passes and battery technology is embraced, research into battery technology will accelerate, leading to significant advancements as EV manufacturers look to source batteries which are both lighter and demonstrate longer battery life. This can be expected to have a major impact on both the minerals used and the proportions in which they are used, in EV batteries.
Notwithstanding this demand optimism for lithium, resource companies are scrambling to increase supply and we in Western Australia have seen a boom in this part of the resources sector with there now being 5 Western Australia lithium mining operations and numerous other exploration companies progressing with resource definition.
The story for graphite is not dissimilar, other than for the exploration and mining activities being largely focussed in Africa.
Given Western Australia is a major producer of lithium, it is no wonder there is a push for the Government of Western Australia to support downstream processing. As stated in a report prepared for the Association of Mining and Exploration Companies by Future Smart Strategies:
“Governments, industry, and the research sector, must capitalise on this unique, one-in-a-generation confluence of local advantages to value-add. For the foreseeable future, Australia can continue to dominate lithium spodumene mining. Government has frequently referred to downstream processing, and the possibility of adding further value to resources rather than mining and direct shipping out.”
We need to be brave and incentivise industry to develop downstream processing capacity so that we can become a mineral processor to the battery market, not just a miner.
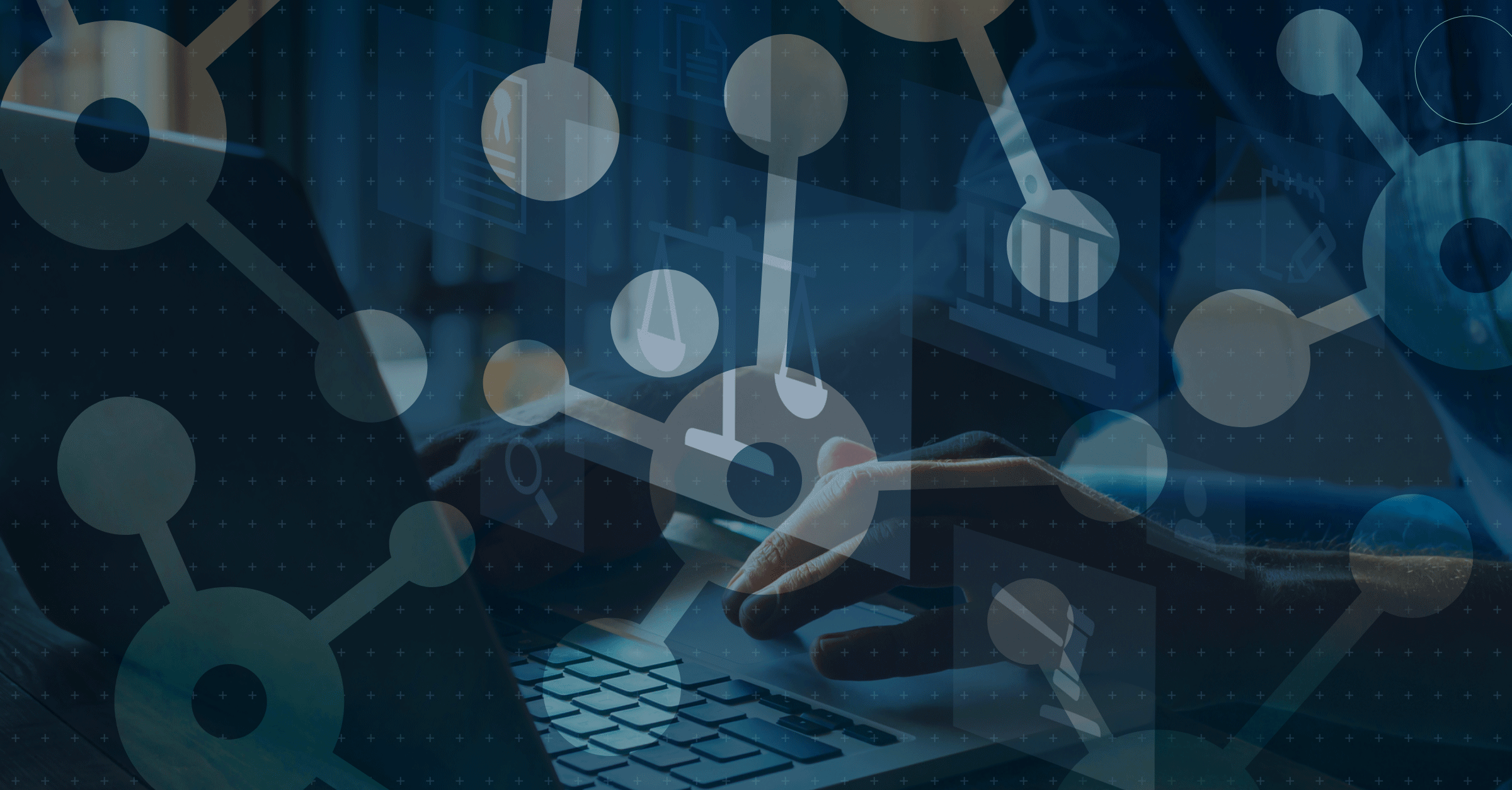
Visit SmartCounsel